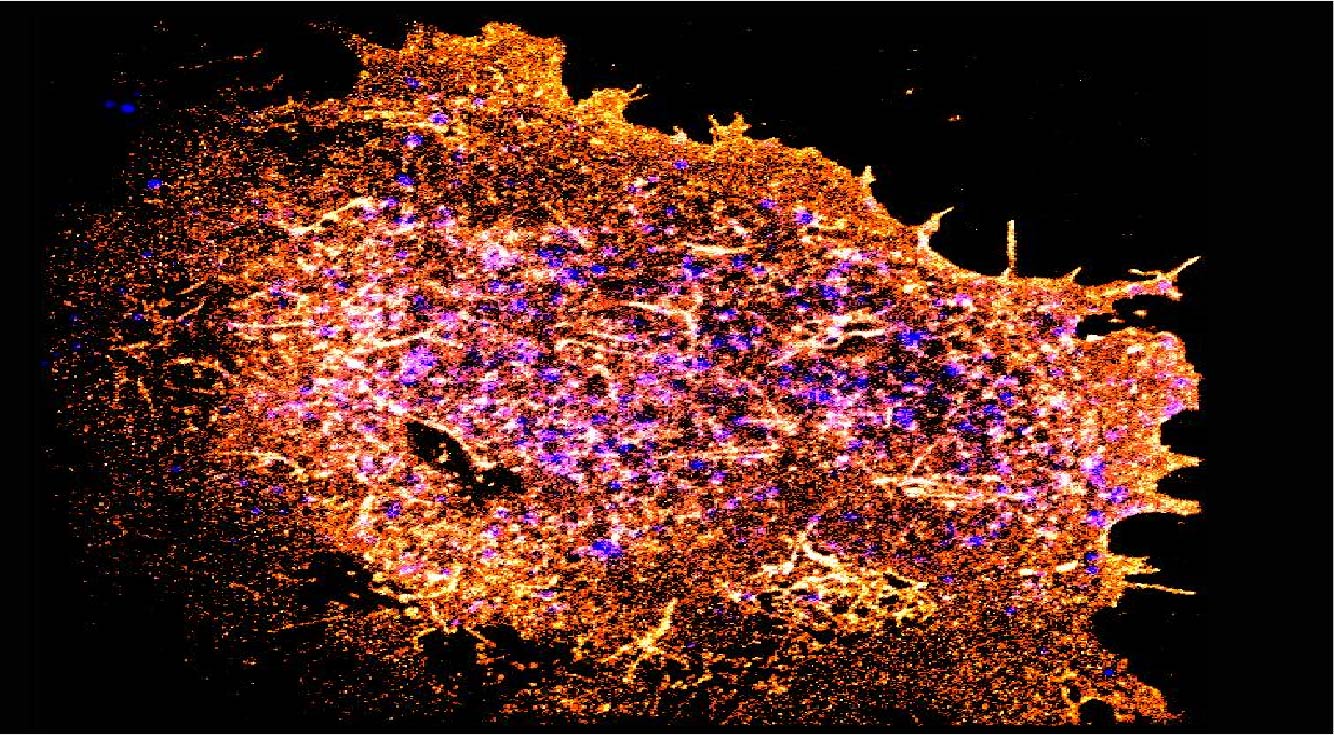
Understanding responsive mechanisms of metalloproteins is key to elucidate biological functions of copper (Cu) and to identify the causes of diseases resulting from abnormal metal homeostasis. The cellular Cu uptake and secretion require relevant metalloproteins to adjust in a spatiotemporally coordinated manner to assure proper cellular Cu level. However, in the Cu field, little is known about how metalloproteins are individually regulated nor systematically cooperate with each other in their native environment, i.e., in cells. Our research goal is to understand the responsive mechanisms of Cu-uptake and secretory metalloproteins in human neurons, with specific focuses on how metalloproteins adjust their behaviors such as spatial distributions, oligomeric states, inter-protein and inter-domain interactions for proper Cu balance in a spatiotemporally defined manner.
Using CTR1 and ATOX1-ATP7A/B as the initial examples of uptake and secretory metalloproteins, our lab aims to (1) quantify Cu-dependent oligomeric state distribution and identify the Cu-responsive moiety of CTR1; (2) define the preferential interaction of ATOX1 to ATP7A and ATP7B and how mutations in ATP7B affect Cu homeostasis in cellular Cu defending. The comparison between hESC-derived healthy and diseased neurons will inform how disease mutations disrupt cellular Cu balance.